Review by Francois Taute
The Skin – Beyond the Barrier
The skin comprises±1.8m2, typically regarded as having a barrier to protect against environmental and xenobiological insults. However, skin is an exquisitely complex sensory and excretory organ [1, 2] – composed of adipose tissue, collagen, keratin, muscle fibers, nerves, and vasculature – sensing circadian changes [1, 3] as well as other environmental conditions (e.g. moisture, temperature), acting as a host substrate for a complex microbiome [4]. The anatomy (Fig. 1) of the skin shows the complexity which constitutes a multipurpose organ, which provides sensitivity to various stimuli (e.g. mechanical, electromagnetic, thermal, and moisture) and can influence mental as well as physical wellbeing.
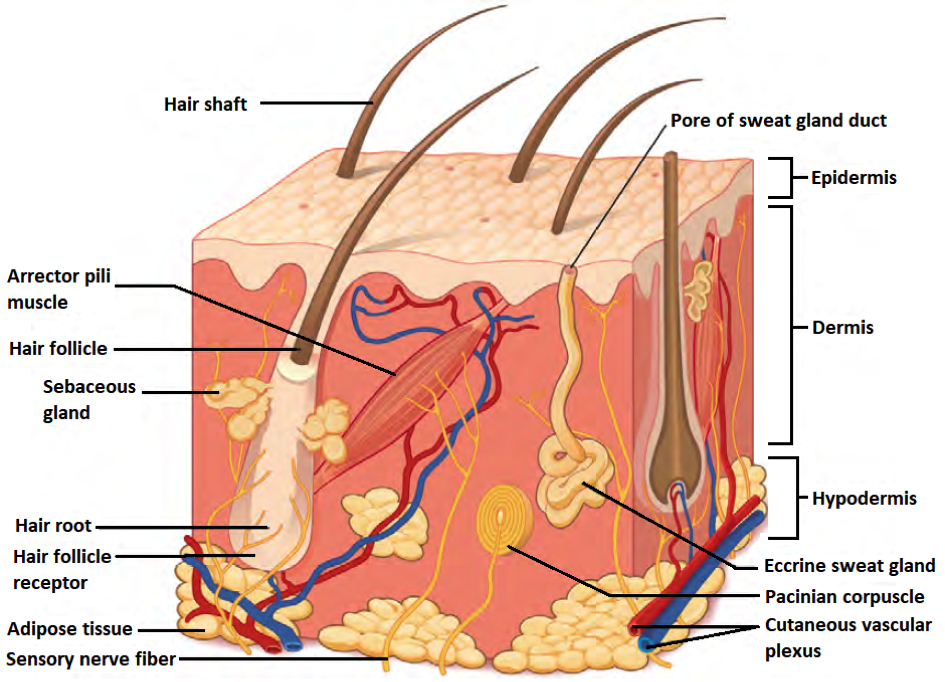
The complexity of the skin also makes it prone to various diseases such as cancer, immunological disorders, inflammation, connective tissue disorders, and infections [5]. Basic and translational science aims to provide answers to improve the understanding of this organ and associated pathologies for improved clinical care. Drug delivery research aims to deliver therapeutic formulations transdermal by diffusion [6] or microneedle injection [7], where wearable biosensors can detect metabolic flux in real-time from the skin [8, 9]. Additionally, the skin (and hair) is an important target for the cosmeceutical industry topical cosmetic and anti-aging applications.
In vitro Culturing of Patient Derived Skin Biopsies Derived In vitro Models
Current in vitro models (2D and 3D) do not always fully recapitulate the complexity of the skin, wherein vivo animal models are becoming less ethically acceptable, especially in the push of the 3R (Replace-Reduce-Refine) initiative). Sufficiently complex in vitro models require more advanced approaches and maximal use of available biological materials.
Typically, a patient skin biopsy will be done when a clinician suspects a pathology. The methodology varies depending on the suspected pathology – a shave-, punch- or excisional biopsy – which will be used for histological analysis and diagnosis. Pathologies (e.g. melanoma) which require complex deep tissue histological analysis will rely on a circular punch-biopsy (1mm < diameter < 10mm). Advancement in tissue culturing methodologies enables skin biopsies [11, 15] and biopsy-derived cells [12, 13, 14] to be cultivated in vitro model (transwell based), providing more physiological as well as genotype-phenotype relevant research models. The ideal skin research model should take the following factors into consideration to recapitulate a physiologically relevant model (Fig. 1):
- Provide a barrier function and resilience to injury and ambient conditions in attempts to recapitulate an air-liquid interface;
- Have histological relevancy with cell layering and sheeting with the inclusion of blood perfusion and innervation; and
- Allow for the incorporation of immune function; and
- Establish oxygen gradients.
The direct use of patient biopsy-derived tissues provides a complex physiological environment that is immune-competent, but the ethical and administrative aspects to obtain this material is inconvenient. Commercial suppliers of skin biopsies are available [16], which retain their immunocompetent status for up to 7 days of culturing in static conditions. The direct use of skin biopsy tissue for an in vitro model with as much tissue intact as possible can provide valuable adipose-associated endocrine/paracrine [17] function and intact native microvasculature, as well as hair and follicles. In vitro models such as 3D tissue engineering [14, 18]; microfluidics and organ-on-chip approaches [19, 20]; patient-derived skin equivalents, and patient-derived xenografts [12] have provided limited complexity models. These reductionist models are suitable for a specific purpose but lack the complexity required to fully comply with the 3R approach and require significant labor-intensive techniques to produce a viable model. Table 1 provides a generalized summary of models made with patient-derived skin biopsies.
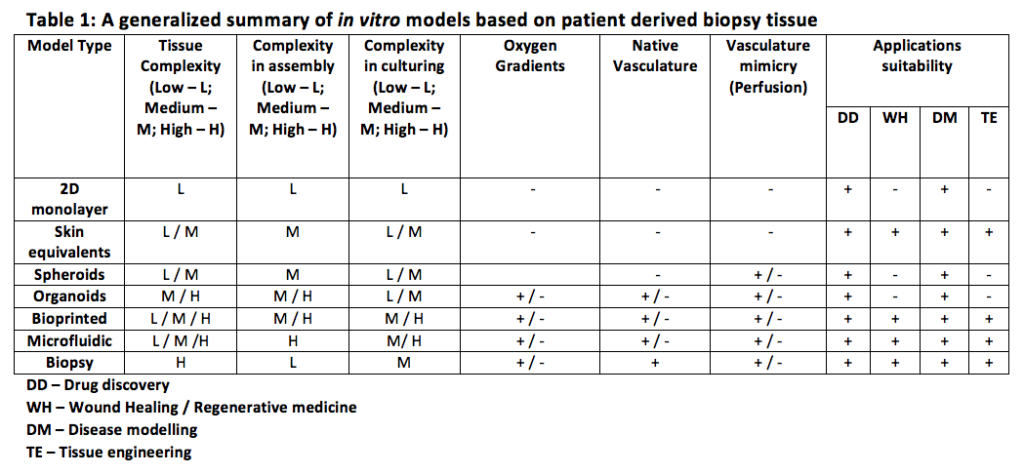
Corzo-Leon et al [15] demonstrated the use of 1cm2human biopsy tissue cultured in static conditions (37°C, 5% CO2) to model healthy (Fig. 2a) and a topical fungal infection (Fig. 2b) over 14 days. The simplicity in the model design provides for minimal processing of samples, where the tissue was cultivated on a small porous platform and maintained the air-liquid interface (Fig. 2c) with histological analysis for apoptosis and anatomy on day 14 (Fig. 2d).
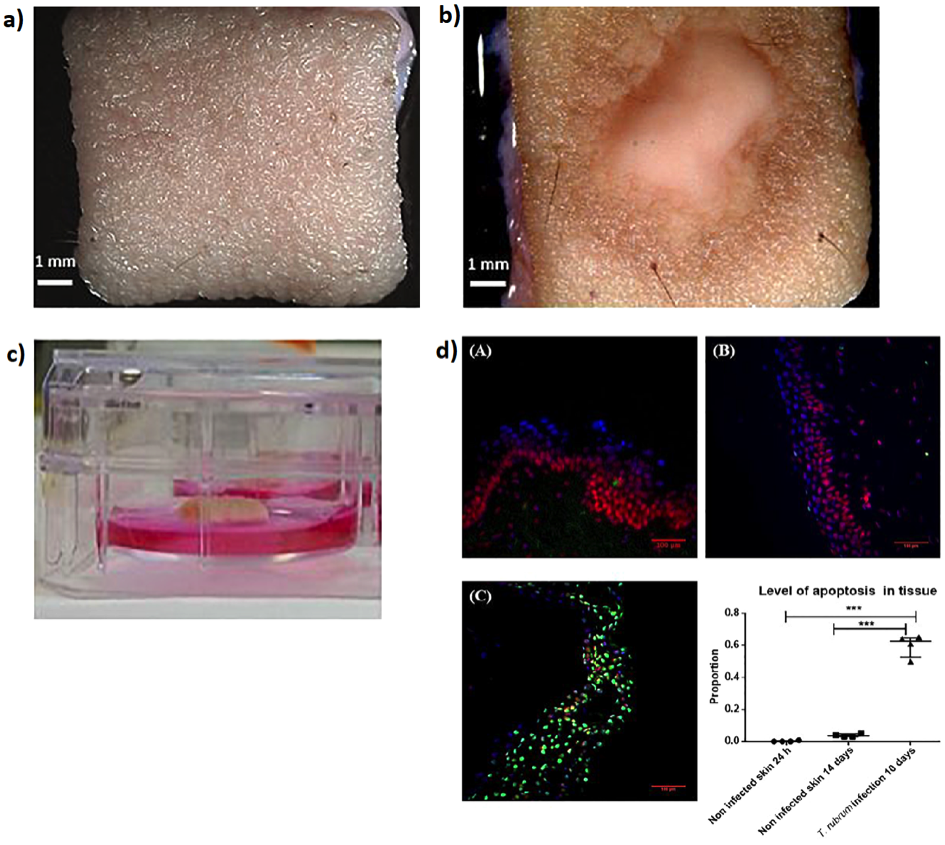
In vitro Culturing of Patient Derived Skin Biopsy Derived In vitro Models
The generation of oxygen gradients is crucial for physiological mimicry of skin in ex vivo-to-in vitro approaches, where Evans et al [ref] showed that the in vivo oxygen gradient can vary from 7 mmHg (dermis) to 0.1 mmHg (sebaceous glands/hair follicles). The oxygen gradients are responsible for biochemical homeostasis [22, 23], wound healing [22, 23], and sustaining microbiota [24, 25] essential for proper skin function. Hypoxia-inducible factor 1-alpha (HIF1α) has been identified as the main regulator (Scheme 1) for oxygen-dependent biology in the skin [22, 23, 26). This is an important consideration as typical culture conditions do not allow for establishing oxygen gradients in in vitro skin models.
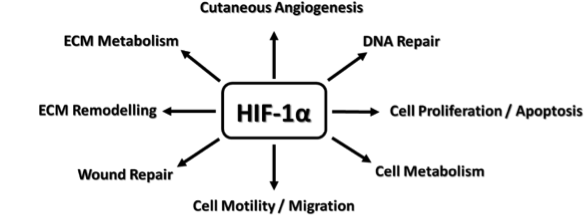
Mieremet et al [27] demonstrated using 2D and 3D skin equivalent models the difference in using normoxia (20% O2) and hypoxia (3% O2) conditions, that under hypoxic conditions the in vitro models histologically compared more favorable to native human skin histology (Fig. 3). Summarized, hypoxia culturing conditions resulted in (i) decreased epidermal thickness in full-thickness models; (ii) altered metabolism and lipid synthesis; and (iii) more humanized epidermal morphogenesis.
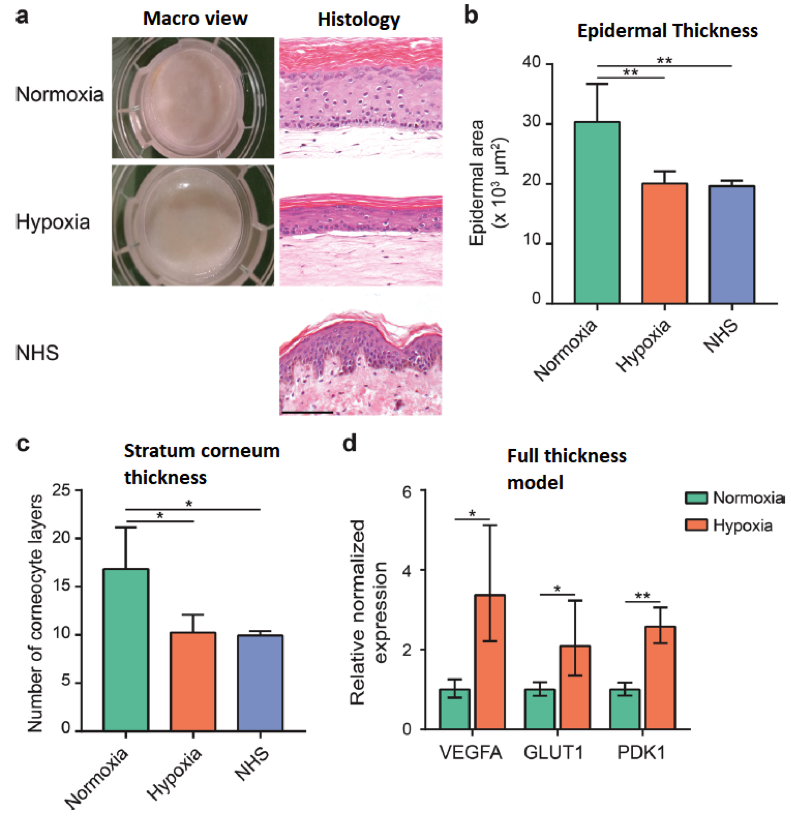
The culture duration for typical static culturing conditions for ex vivo skin biopsies are 10-14 days [11, 14, 27], where other methodologies to be considered for improved culturing and more physiologically relevant in vitro skin models are to optimize the cell culture media formulation [28] or the use perfusion systems [29], where culturing was sustained for up to 75 days for the former and improved histology attributed to shear stress for the latter. Skin biopsies
The choice of in vitro culturing methodologies for ex vivo patient tissue is dependent on the intended application where the researcher must answer the following questions:
- What pathology do I aim to recreate and what phenotypes need to be maintained;
- The sampling methodology and duration required to fully recapitulate a single therapeutic dose VS acute multiple therapeutic intervention;
- The in vitro methodologies as pertaining to culture media composition and physicochemical homeostasis (e.g. temperature, oxygen, ambient light, etc.);
- The sensitivity of the cell/tissue type to handling frequency, temperature variation and gas gradients; and
- Are real-time investigative methods (e.g. microscopy, impedance) or endpoint analysis (e.g. histology) required? Skin Biopsies
In the era of precision approaches to provide individualized therapeutic solutions will ultimately de dictated by consensus: How accurate does a preclinical or clinical model must be to recapitulate “good enough” physiological and biochemical parameters to find a solution in a time-appropriate manner.
References
- OpenStax College, Anatomy & Physiology. OpenStax College. 25 April 2013.
- Kazem, S. et al.2019. Skin Metabolism Phase I and Phase II Enzymes in Native and Reconstructed Human Skin: A Short Review. Drug Discovery Today, 24(9):1899-1910.
- Yamaguchi, A. et al. 2017. A Simple Method Using Ex Vivo Culture of Hair Follicle Tissue to Investigate Intrinsic Circadian Characteristics in Humans. Scientific Report, 7:6824.
- Byrd, A.L. et al.2018. The Human Skin Microbiome. Nature Review Microbiology, 16:143-155.
- Chiang, N.Y. and Verbov, J. 2ndEdition (2014). Dermatology: A Handbook for Medical Students and Junior Doctors. British Association of Dermatologists.
- Kadam, A.S. et al. 2014. Transdermal Drug Delivery: An Overview. International Journal of Research and Development in Pharmacy and Life Sciences, 3(4):1042-1053.
- Waghule, T. et al.2019. Microneedles: A Smart Approachand Increasing Potential for Transdermal Drug Delivery System. Biomedicine & Pharmacotherapy, 109(2019):1249-1258.
- Kim, J. et al. 2019. Wearable Biosensors for Healthcare Monitoring. Nature Biotechnology, DOI:10.1038/s41587-019-0045-y.
- Chung, M. et al. 2019. Wearable Flexible Sweat Sensors for Healthcare Monitoring: A Review. J. R. Soc. Interface, 16:20190217.
10.http://eara.eu/en/the-3rs-principles/
- Sidgwick, G.P. et al. 2016. Functional Testing of Topical Skin Formulations using an Optimised Ex Vivo Organ Culture Model. Arch. Dermatol. Res., 308:297-308.
- Florenes, V.A., et al. 2019. A Three-Dimensional Ex Vivo Viability Assay Reveals Strong Correlation Between Responses to Targeted Inhibitors and Mutation Status in Melanoma Lymph Node Metastases. Translational Oncology, 12(7):951-958.
- Groeber, F. et al.2016. A First Vascularized Skin Equivalent as an Alternative to Animal Experimentation. ALTEX, 33(4):415-422.
- Schimek, K., et al. 2018. Bioengineering of a Full-Thickness Skin Equivalent in a 96-Well Insert Format for Substance Permeation Studies and Organ-on-Chip Applications. Bioengineering, 5(43):doi:10.3390.
- Corzo-Leon, D.E., et al. 2019. An Ex Vivo Human Skin Modelto Study Superficial Fungal Infections. Frontiers in Microbiology, 10:1172.
16.https://www.genoskin.com/en/human-skin-response/
- Park, J. et al.2011. Paracrine and Endocrine Effects ofAdipose Tissue on Cancer Development and Progression. Endocr. Rev., 32(4):550-570.
- Klicks, J. et al. 2017. In vitroSkin Three-Dimensional Models and Their Applications. Journal od Cellular Biotechnology, 3(2017):21-39.
19.Sriram, G., et al. 2018. Full-Thickness Human Skin-on-Chip with Enhanced Epidermal Morphogenesis and Barrier Function. Materials Today, 21(4):326-340.
20.Terry, J. and Neethirajan, S. 2014. A Novel Microfluidic Wound Model for Testing Antimicrobial Agents against Stahphylococcus pseudintermedius Biofilms. Journal of Nanobiotechnology, 2014, 12:1.
- Evans, N.T.S. and Naylor, P.F.D. 1967. The Oxygen Tension Gradient Across Human Epidermis. Respiration Physiology, 3(1):38-42.
- Rezvani, H.R. et al. 2011. HIF-1αin Epidermis: Oxygen Sensing, Cutaneous Angiogenesis, Cancer, and Non-Cancer Disorders. Journal of Investigative Dermatology, 131:1793-1805.
- Rezvani, H.R. et al. 2011. Loss of Epidermal Hypoxia-Inducible Factor-1αAccelerates Epidermal Aging and Affects Re-Epithelialization in Human and Mouse. Journal of Cell Science, 124:4172-4183.
- Chen, Y.E. et al. 2018. Skin Microbiota-Host Interactions. Nature, 555(7697) :427-436.
- Zeng, B. et al.2017. High-Altitude Living Shapes the Skin Microbiome in Humans and Pigs. Front. Microbiol., 8:1929.
- Cowburn, A.S., et al. 2013. HIF Isoforms in the Skin Differentially Regulate Systemic Arterial Pressure. PNAS, 110(43):17570-17575.
- Mieremet, A., et al. 2019. Human Skin Equivalents Cultured under Hypoxia Display Enhanced Epidermal Morphogenesis and Lipid Barrier Formation. Scientific Reports, 9:7811.
- Andrade, T.A., et al. 2015. Ex Vivo Model of Human Skin (hOSEC) as Alternative to Animal use for Cosmetic Tests. Procedia Engineering, 110(2015):67-73.
- Struver, K. et al.2018. Development of a Perfusion Platform for Dynamic Cultivation of In vitroSkin Models. Skin Pharmacol. Physiol., 30:180-189.